E. Home Heliostats
E1. Heliostats
A heliostat is a solar tracking mirror. As the sun moves across the sky it reflects sunlight onto one spot all day, onto one solar target. Even while clouds are temporarily hiding the sun, the heliostat uses its motors to rotate its mirror from left to right, also up and down as needed, to always bounce sunlight onto its target whenever the sun peeks through the clouds.
In my own world of heliostats the use of a single heliostat differs from industrial-strength sunlight collection. I'll start by discussing single heliostats because I want to complete my discussion on heating buildings.
The Genzyme Center in Cambridge, MA, built in 2003, uses multiple heliostats to reflect sunlight into the building. Employees like the results, with the understanding that employee polls might be biased in favor of their own company.
I'll repeat a few cautions: no one should ever stare at the sun directly because it can cause eye damage. I've heard one recommendation that even 6 times normal solar concentration is getting into a danger zone because heat buildup can lead to melting and fire hazards. For home use, 4 times normal concentration should be safe if you have 100% assurance that your reflectors are flat and not bowed inward by the wind. As a practical safety measure I have purchased my eyeglasses with a protective UV coating, and I would recommend this precaution for any solar experimenters or builders.
E2. Combining a heliostat with a skylight
Skylights use mirrored solar tubes to bring sunlight down from a skylight through a wide attic crawlspace or through thick roof insulation into a room.
I want to combine a heliostat with a mirrored tube. For home use I'd recommend a heliostat mirror with four flat rectangles within a larger rectangle, where all four surfaces are aimed at a window at solar noon on February 21, 1/4 of the way between the December 21 winter solstice and the June 21 summer solstice. As with my greenhouse's optics, keeping the heliostat as close to the entrance of the mirrored tube as possible is going to reduce the percentage of light that misses the target.
Because the sun moves 246 degrees from northeast to south to northwest each day in June, but the sun only rises from the horizon at dawn to about 72 degrees above the horizon at noon (all this at 42 degrees latitude), four vertically oriented or portrait rectangles will see less focal distortion than four horizontally oriented or landscape rectangles.
As discussed elsewhere, with mirrored tubes, multiple passive solar targets are an option for notably better heliostat focusing.
The heliostat mirror's rectangles need to be sized to the targets. When the heliostat mirror is taking sunlight coming in from due west and reflecting it due south, remember that the mirrors need to be 1.414 times as wide as the target due to the 45 degree reflection angle. It's probably better to throw away a bit of extra sun at noon than to have differences in sunlight strength between solar noon and 6:00 p.m.
As with the greenhouse reflector wall, often the best place for a heliostat is on top of a pole. As with the greenhouse, I generally prefer to have the heliostat relatively close to the target for an easier focus with less distortion at steep angles.
Even houses that are 80% covered by tall trees often have some branch-free corridor for reflecting sunlight down to the second floor north wall of the house. With a larger housing complex, heliostats mounted on nearby houses can handle the reflection job. Specially built houses can have heliostats mounted on their own roofs.
It's often better for the solar window to be well above ground level for safety. An eave over the horizontally facing window will keep most precipitation off of the window, just as eaves over most normal windows keep most precipitation off.
A friend recently had a cracking problem with his clear skylight. Wall windows don't have the various roof leakage and large hail problems that plague skylights.
From there the incoming sunlight might be inexpensively reflected 90 degrees downward with a mirror mounted at a 45 degree angle to the window. The light can travel rather directly down a mirrored tube to a room, say, in a house's basement. The four incoming sunlight reflections will be coming from slightly different angles but the incoming rays will all be almost parallel to each other. Any sunlight bouncing against any of the mirrored tube walls will be bouncing at a sharply oblique angle. Illumination losses from sunlight bouncing obliquely against the mirrored walls will be quite low.
If four large square heliostat mirrors are all reflecting on one target, the four merging beams of light might be converging at, for argument's sake, 5 degrees apart from each other. Inside the mirrored tube the four light beams would be diverging at 5 degrees from each other. Given a perfectly straight mirrored tube, or given reflecting mirrors that turn all four of the beams of light at a certain reflecting angle, only tiny edges of the incoming light beam are going to touch the mirrored walls at a highly oblique 1 degree angle and reflect back into the light beam at an equally oblique angle. We have nearly parallel concentrated sunlight in the mirrored tube. We can safely ship concentrated sunlight down a tube within, say, a five story tenement to the basement. In the basement we can convert the solar heat into hot water, store the heat for winter night heating needs, grow some plants or simply enjoy some sunlight.
For house retrofits it's possible to build a mirrored tube down the outside wall of a house and then refocus all of the incoming near-parallel sunlight through an existing casement window space into the basement.
E3. A mirrored window screen concept for a hurricane-proof heliostat
I once saw an architectural study of wind uplift on the eaves of a building's roof. The architect removed 10% of the eaves in a regular pattern, leaving air holes for the wind to pass upwards. The holes reduced uplift on the eaves by about 40%. People who carry banners in parades also understand the advantages of cutting regular air holes in the banners to spill wind.
Window screens on enclosed porches can survive some pretty strong wind gusts. That's because wind overpressures don't build up on one side of a porch window screen. A converse example would be a sail on a sailboat where zero air gets through and the sail builds up a strong air pressure differential which pushes the boat forward. I've seen a traditional Brazilian sailboat with a woven cloth sail, where the sailor must regularly splash water on the sail to make the boat travel faster. The water closes the many air holes between adjacent threads on the cloth sail. In this way the water increases the wind gust pressure on one side of the sail. The closing of millions of tiny air holes with water makes the boat move faster, so that local people always wet the boat's sail before and during use.
Existing building roofs are often weak. In general we want maximized solar reflections while minimizing hurricane-level wind gust forces on the building's roof. One solution is to design a flat heliostat mirror with a vast number of tiny air holes, a window screen of a mirror. We should be able to reflect 90% of the incoming sunlight while at the same time reducing the wind load on the heliostat and on the roof by at least 50% or perhaps far better.
E4. A house greenhouse
The conservatory is one of the nine mansion rooms in the game “Clue”, as in, “Mr. Green with the lead pipe in the conservatory.” A plant conservatory would be an enjoyable luxury room found in a decent mansion. Lots of living houseplants humidify the house's air. A spider plant can capture formaldehyde molecules out of an airtight home's atmosphere.
One of my greenhouses without the greenery would still be a house, a wonderfully warm and sunny house, a premium house on a sunny February afternoon. We found this out with the Attleboro greenhouse. My construction partner's house was 68 degrees and dark, while the greenhouse on a good February afternoon was often in the high 70s and flooded with bright midwinter sunlight. On a good afternoon he would move in.
From a lower middle class financial viewpoint a bare bones starter house needs to save the homeowner's money over the years. Solar heat can save fuel. Even an infinite amount of home insulation can't completely handle a winter day when the front door is left propped open, but solar heat with short-term heat storage might be a great partial solution to the open front door problem.
E5. A basement greenhouse
The concept of a rooftop greenhouse has fundamental problems. Thermal storage belongs in the basement. Rooftop windows don't insulate well on winter nights. The rooftop would have to support extra tons of topsoil. Garden hose spills in the greenhouse might drip through the floor and then down through somebody else's ceiling.
If a strongly concentrated source of sunshine can be piped through a mirrored duct into a basement, the basement might turn out to be an excellent place for a winter greenhouse. Winter sunlight creates winter heat and then excess winter sunny day heat is stored within thermal mass (the topsoil) or in the center of the basement's concrete floor. A basement greenhouse doesn't have window problems. A plant watering malfunction can flood a concrete basement with relatively little structural damage to the house. Great quantities of topsoil will be heavy, but concrete basements handle heavy loads better than rooftops.
Slightly overheating a basement on a winter day is usually no problem because basements are often used for the storage of goods. At the right sunny hour, a notably warm basement room would be a desirable place for private winter sunbathing, caring for an infant or a hot yoga session.
One big side issue: the architect of such a basement greenhouse must carefully regulate the maximum humidity in the basement. Garden plants create humidity. Given long-term excessive humidity with condensation, the house's floor joists can potentially rot away.
One solution on a winter day is a fresh air exchanger. Winter air can be extremely dry,
E6. A cafe sun spot
I've seen coffeehouses constructed with rather fake-looking natural gas fireplaces. A warm, sunny sun spot in a coffeehouse is likely to meet with greater customer satisfaction for a number of good reasons. People love natural sunlight, especially when they can't get it elsewhere. Natural sun produces Vitamin D. It wards off Seasonal Affective Disorder. It's invitingly warm on a cold winter day. People pay tanning salons quite a bit of money for a certain suntanned look. The business owner can grow lush-looking natural houseplants all winter in a sun spot. In July the heliostat can be repointed away from the sun to turn off the sunlight effect. Customers appreciate a gas-free environment with lowered or zero fossil fuel use, and the owner pays lower gas and electric bills.
Every Frost Belt office building should have a lounge, a water cooler area or a nap room with a winter sun spot. First grade open classrooms should have sun spots with small nature labs. Frost Belt retirement homes should have winter sun spots because retirees feel better in Florida during the winter because of the heat. Also, retirees with poor vision can read and retirees can productively grow plants for fun or for a small profit.
I've seen attempts at daylighting big box stores with skylights, where a few skylights were considered inadequate to light the store. In the end the store was flooded with fluorescent lighting. A combination of a tracking heliostat and mirrored tubes carrying sunlight concentrated to 400% of normal sun will make it possible to more strongly daylight interior rooms. The beauty of using a solar tracking heliostat is that the strength of interior sunlight near sunset can be almost as strong as noontime sunshine.
Special photosensitive lighting systems will be installed to compensate for in-and-out cloudy periods.
On the hottest July days, laying an infrared and ultraviolet ray filter over the target window will reduce the building's cooling costs. Removing the infrared filter for winter should reduce the building's winter heating fuel costs.
It's still possible to put photocells next to the solar target so that the heliostat's motors know when to turn the mirror, and it's possible that the heliostat's software already knows where to point its mirror. I'd combine both of these aiming tools.
E7. A garden surrounded by skyscrapers
In the shade of other skyscrapers or in the shade of trees, gardens won't grow anything. A heliostat with a single flat mirror leaning over the edge of a tall building can bounce many hours of constant daylight down to a ground level garden spot next to the building. The garden spot can be otherwise shaded by buildings, but it would have sunshine all day for good vegetable growth.
It's possible to have six months of urban garden growing in the shadow of tall buildings, and then another six months of heliostat winter heating.
E8. Solar assists for clearing of snow
As I write this sentence an 18 inch blizzard is setting up. The nearby ocean is about 2 degrees Celsius too hot, and so a snowstorm is about to bomb out. After several months of no sun at all the polar air is just as cold as ever, but climate-enhanced storms are stronger and better able to pull the polar air down into place.
I live in a moderate Frost Belt climate where reflecting 4x sunshine down onto a blacktop driveway or sidewalk might melt away the snow on a day when the temperature is nearly freezing. This use of a heliostat would require a camera able to see blacktop and white snow. Computer software would remember which spot to melt first and which path to melt first. If a camera detects any motion, it's best practice that the heliostat move its solar heat ray away from the driveway.
E9. Industrial heliostat field construction
I propose that we develop a source of low-cost, on-demand high temperature heat. High temperature geothermal heat storage can create steam on demand to power an electric turbine when wind and solar are both not meeting electric grid demand. Heat stored at industrial-strength temperatures can also heat districts in winter and perform industrial heating jobs.
The previous idea of reflecting slightly concentrated sunlight straight (or relatively close to straight) down a mirrored tube can be applied to a field of heliostats. I'll be examining the various limits of what can be done in order to make a more effective heliostat field. Here's what I want to discover:
- How nearly parallel we can keep the sunlight in the mirrored tubes?
- How easily can we bend mirrored tubes around corners without losing much of the power of polarized light to oblique reflectance? Otherwise, how can we maximize sunlight delivery with a series of non-oblique reflective mirrors?
- Can we save money by merging and combining mirrored tubes full of concentrated light?
- How strongly can we safely concentrate the sunlight within these tubes.
- Can we exclude all birds from the tubes?
E10. Minimizing cosine focusing errors
They say that even a stopped clock is right twice a day. By the same standards a sunlight-focusing heliostat concentrates light with a perfect focus twice a year. The rest of the time, sometimes the left edge of the heliostat mirror is positioned just a bit too far back and the right edge is a bit too far forward, or the top edge is tipped a bit too far back and the bottom edge is a bit too close for an accurate focus. Sometimes sun reflected from the edges of a curved, concentrating heliostat or linear trough will miss the target by just a little bit, sometimes by a lot more. We want to keep these cosine focusing errors somewhat under control by not having outrageously turned-around heliostats.
E11. Building multiple reflecting targets for each heliostat
If the heliostat has to concentrate 16 hours of sun onto one target, it needs to rotate 60 degrees to the east or to the west in order to pick up 4:00 a.m. sunlight at dawn or 8:00 p.m. sun at dusk. Keeping a concentrating focus while turned 60 degree just isn't possible. Worse, in the land of the midnight sun 24 continuous hours of daylight might be arriving from various directions, north, south, east or west.
One solution is to install more than one solar target for each heliostat. Heliostats are most efficient, and the incoming light is most close to parallel, when they are focused almost straight toward the sun. I'll make a rough assumption that four solar targets for each heliostat, one target every 60 degrees in a ring around each heliostat, will be optimal at 45 degrees north latitude. Six solar targets in a ring might be optimal well above the Arctic Circle. Installing eight targets around each heliostat might lead to extreme focusing accuracy, which the system probably won't need, but increasing the number of targets adds to the system's cost.
All four targets are more or less aimed for a March 21 solar elevation. The target for 6:00 am collection is low, 10:00 am is high, 2:00 pm is high and 6:00 pm is low.
In truth the early morning and late afternoon targets might be aimed for April 21 sun, because at march 21 the sun sets at 6:00. Whenever the sun is less than 20 degrees above the horizon, almost every target on the heliostat field is seriously shaded by the heliostats in front of it. Sunlight near the horizon can also be shaded at dawn by low clouds and by the tops of nearby tall trees. In winter, early evening solar tracking is moot because sunset takes place around 4:00 p.m.
If we only get 12 hours of truly usable sunlight in June and less by August, never mind December, the four heliostat targets can be profitably positioned a bit closer than 4 hours apart from each other. Let's try setting their exact focus points at around 8:00 a.m., 10:30, 1:30 and 4:00. This leaves no more than 3 hours of aiming between the two mid-day targets. With a maximum three hour coverage period the sun tracks 45 degrees across the horizon. For each target the moving sun would be a maximum of 22.5 degrees off of straight-on, either to the left or right, and so the mirror might be turned 11.25 degrees to the left or the right at the extreme. Turning the mirror a maximum of 11.25 degrees off of straight delivers a low cosine of azimuth (turning 360 degrees around the horizon) focusing error.
The heliostat's maximum cosine focusing error in the elevation direction is also well manageable. At 45 degrees north on March 21 at noon, the sun is 45 degrees above the horizon. On June 21 it's 23.5 degrees higher than on March 21. On December 21 it's 23.5 degrees lower. At worst, the heliostat has to pivot 11.75 degrees higher than straight-on in June or 11.75 degrees lower than straight-on in December to hit its target.
E12. Distance from the heliostat to the solar target
Heliostats used in solar power towers sometimes reflect sunlight toward a target hundreds of meters away. This focusing distance requires precision parts for precision focusing. Because I use mirrored tubes, my solar targets are perhaps 5 meters away. The short focusing distance solves focusing errors. It makes the system work like a charm and it saves the developer some serious money.
E13. Hexagonal heliostat fields
A hexagonal array of heliostats on a field captures more sunlight per hectare than a square array.
Heliostats can be self-contained units. A self-contained heliostat with its own on-board battery and small photovoltaic panel won't need any electric wires. Optimally we want to assemble each heliostat onsite from modular, replaceable parts, anchor it to the ground, turn it on and then the heliostat automatically finds its closest target or targets.
I'm penciling in a standard solar reflecting mirror almost 6 meters wide and 3 meters high, aiming sunlight into a solar target placed below the bottom edge of a nearby reflecting mirror. The front of the solar target is 2 meters wide by 1 meter high, 1/9 of the size of the reflecting mirror.
Beyond the front edge of the solar target is a mirrored tube that carries the concentrated sunlight away. Typically the concentrated sunlight will heat some target at the other end of the tube. Photovoltaic power and daylighting are alternative uses.
- - - -
I assume 6 meter by 4 meter windowscreen reflectors, 1/20 of the area is airholes, about 5 mm apart in a hex formation. I assume 9 insect eyes and 4x concentration.
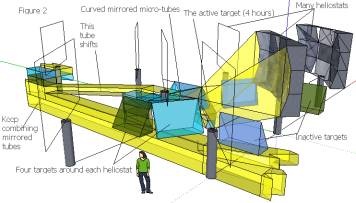
The next reflector up is also 6m x 4m. at 10 degrees above the horizon at 3:00 pm on december 21 both reflectors are tipped forward, which means the back end is tipped backward if it rotates on the middle. the target has to be down at 5.5 m away from the reflector's post and just below the post. This leaves 0.5 m.
We would build four individual reflectors, the left and right high, and the left and right low, for any particular latitude. Our replacement bug eye modules would snap into our reflector frames.
At the entrance to each solar target we need a grill in order to exclude all birds and bats. A thin wire grill might work.
E14. Sending near-parallel sunlight through tubes and around bends
A fiber optic cable contains thin, flexible glass fibers. Laser light put into one end of a glass fiber will bounce against the walls of the curving glass fiber until the light comes out the other end, sometimes 20 miles away. The thinness of the fiber relative to the gentle bends in the fiber determines how well parallel light stays within the fiber.
I don't perfectly understand the physics of oblique reflection of light but I know what I want. I want to be able to reflect useful amounts of solar-sourced heat down a flexible pipe. A flexible pipe would be easy to thread through tight spaces and through curves in an existing house. So, I want to at least ask the right questions:
- Do we need certain light wavelengths to optimally bounce against the reflective sides of a glass fiber?
- Can we use wider pipes filled with air?
-Would a wider flexible pipe filled with pure water work? Do we need to eliminate all oxygen from the water in order to not degrade the reflecting walls of the pipe over time? Will another fluid work within the light pipe?
- Can we get near-parallel sunlight down the pipe? How close to parallel light do we need? How much energy loss will we see?
Unfortunately, light polarizes (and most likely loses some energy) with the first oblique bounce and again with the second oblique bounce. We're best off bouncing nearly parallel sunlight off of non-oblique mirrors within a mirrored tube. I still want to discover how laser light can bounce obliquely off of the sides of glass fibers over a 10 mile distance without losing signal.
Converting sunlight to laser light might be energy-ineffective, but sunlight at the source is terribly cheap. For the cost of an acre of land, sunlight can be collected forever.
E14a. Fitting almost-parallel sunlight into a tube
If I'm going to be working with almost parallel sunlight traveling down a mirrored solar tube, I want to know the theoretical maximum amount of near-parallel sunlight that I can cram into a 1 meter circle.
As viewed from the earth's surface, the sun is a disk in the sky with an average width of 0.535 degrees. An absolutely perfect telescope mirror can focus all of its reflected sunlight onto a 1 meter diameter disk, for an excellent detailed image of the sun on the disk, if the telescope mirror is about 107 meters away from the target disk.
In this drawing I'm assuming that the light can arrive on the target disk no more than 5 degrees off of straight. The large telescope reflecting mirror on the far end is 9.37 meters in radius and concentrates 88 suns onto the target. If we can get by with 10 degrees off of straight, the theoretical maximum power on the one square meter target is 356 suns.
This calculation also tells us that if we have an oval-shaped hill slope solar collector equivalent to 100 meters in vertical elevation by 100 meters wide, we can fully hit that target with any number of flat heliostats at a range of 10.7 kilometers away from the target, or at any closer distance. At a range of 10 kilometers, solar power losses due to low level smog may be a side issue to consider in some locations. Especially but not necessarily if the collector is on the north-facing side of a valley and our heliostats are across the valley on mainly south-facing hillsides, we should be able to safely redirect 5 suns onto each part of the target without overly frying any birds that chance to fly between the heliostats and the collector. Five direct suns on a small target might be ten times more economically efficient than 1 natural sun arriving at the target at an oblique slant near sunset, where the collector would be spread over a proportionally larger area.
Owners of parking lots in overheated climates might want us to reflect some of their unwanted parking lot sunlight away, so as to cool off the cars. As such, a nearby hill slope collector could economically gather solar heat bounced from an urban landscape across a valley, up to ten kilometers away. Such an urban landscape would benefit greatly from relative lack of solar exposure, and local air conditioning costs would be expected to drop.
Because the sun would be arriving at the hillside collector at an oblique angle all day, the 100 meters in elevation collector could be built up the slope as 33 separate rows of vertical collector walls 3 meters tall. If we know that the reflected sun is always arriving within 5 or 10 degrees of being level with the horizon, we can use linear trough mirrors to further concentrate the incoming solar power upon a pipe filled with a eutectic salt mixture or with hot oil.
Except for the bottom row, the front-facing glass walls of these collectors slope downward. 8% of the incoming heat will be reflected away by any glass pane, but that 8% will be re-aimed at the collector just below it for extra heat absorption.
We may not be able to afford a perfect telescope mirror because of many sources of focusing errors, but we at least can calculate the maximum possible sun power that we can send down a tube or a pipe.
The simplest way to bend almost parallel light around a corner is to use a mirror in the tube.
Assuming that a concentration of 30 suns within a light tunnel is optimal, we want to shrink the 2 meter by 1 meter front of the insect eye to a mirrored rectangular light tube 1 meter by 2/3 meter at the back end. The nine insect eye sections will all deliver almost-parallel concentrated sunlight almost directly down the mirrored tube.
E15. Combining mirrored tubes to save mirrors
Mirrored tubes containing concentrated solar rays, all traveling down the tubes within a few degrees of the tubes’ orientation, are combined to make much larger and more economical mirrored tubes. The larger the mirrored tube, the less sunlight bounces off the walls at oblique angles, polarizes and loses energy.
Given a 350 meter wide by 400 meter long field of 6 meter wide by 4 meter tall heliostats, all on 2 meter high posts, the available room for mirrored tunnels between adjacent 2 meter posts is 6 meters wide by 2 meters high. We can fit 36 light tubes that are 1 meter wide by 2/3 meter tall within this space, and so 36 rows of these heliostats will fill the available space. If we raise the heliostats onto 4 meter high pedestals closer to the edge of the field, we can have 72 rows of heliostats pumping the equivalent of 3000% of normal sunlight strength to the edge of the field.
36 individual mirrored tubes 1 meter high by 2/3 meter wide can be combined into one 4 meter high by 3 meter wide mirrored tube. This size mirrored tube can roughly fit between the heliostat posts, noting that in a hexagonal arrangement each row of posts is offset 3 meters from the next row. Such a mirrored tube can be bent to direct concentrated sunlight up and over ridges or other rough terrain as needed, using the same light-bending principles as previously described.
Beyond the last row of heliostat posts on a solar field we can dispense with the walls that separate adjacent mirrored tubes. On a sunny day a wall of perhaps 3000% sunlight, 4 meters high by, say, 400 meters long, can flow out of the side of a heliostat field. We will need to erect thin and mirrored posts within the mirrored tube to support the mirrored tube's ceiling, or else we'll need some type of suspension bridge arrangement to hold up the mirrored ceiling.
For longer distance shipping of concentrated sunlight we might rearrange the mirrored tunnel from a rectangle 4 meters high by 400 meters long to a square 40 meters high by 40 meters long. On a sunny day this square tube would ship as much as 48,000 square meters of direct sunlight, which is stronger than slanted winter sunlight. The roof and floor of this mirrored tube need not be that heavy, and the roof can be supported from above by suspension cables. The tube should be able to leap highways. The tube need not be square if the use of a circular or a hexagonal light transmission tube saves money, or if an oval light tube shape has better high wind gust performance.
A second wall of sunlight can be coming out of the opposite side of the field from 72 other rows on the other side of a target. Such a heliostat field might be 700 meters across because heliostats in a hexagonal arrangement can be packed more densely than in a square arrangement. The rotating heliostat mirrors need to not bump each other's edges as they focus due east, southeast, southwest and due west.
E16. Solar concentration limits within mirrored tubes
I'm taking an engineer's guess that industrial-strength sunlight can be safely concentrated by a factor of 30 within glass mirror-lined mirrored tubes, using long strips of perhaps 1 mm thin and lightweight glass on the walls. It's always possible that we should never dare to go beyond a factor of 10. It's also possible that we can safely achieve a factor of 100 suns worth of near-parallel sunlight. No matter what the concentration factor, we can pipe a serious volume of sunlight for a kilometer or so at a reasonable cost if that's what we need.
Heat buildup within light tunnels will be a problem. A laminar flow of dust-free air, headed toward the solar target, can carry some of each wall's excess heat away, toward where the heat might be put to use. A slight laminar outflow of dust-free air emanating from the insect eye targets would be needed to exclude dust.
I'm taking an engineer's guess that 98% of the light in a mirrored tube will be within 10 degrees of traveling straight down the tube. We might do better or we might not.
Heat in a solar thermal system is guided by five basic principles: heat gain; heat transfer; heat storage; heat transport; and heat insulation. My proposed heliostat system should post outstanding heat gain per acre and remarkable heat transport abilities. Heat transfer, heat storage and heat insulation innovations will be covered in the next chapters.
E17. Tools for absorbing solar heat
At the heat target, straighter light can be more accurately concentrated on a target. We'll be interested in minimizing radiant heat losses at the target, and more nearly parallel light in the tube helps us with this task.
E18. Heliostats cooling parking lots
A large parking lot can be outfitted with poles on concrete bases, so that cars can't easily knock the poles over, and then a field of wind gust spilling heliostats and mirrored tubes can be installed above the parking lot. This would cool the parking lot. A slight amount of light would leak through the micro-holes in the heliostat mirrors to the parking lot, and at noon a rather large amount of light could leak down through the heliostat field into the parking lot. I could see an argument for using somewhat over-height heliostats for capturing noontime sun effectively at summer noontime angles, in order to cool the parking lot.
E19. Meltdowns and hanging roof mirrors
Sooner or later a meteor or an equipment failure will knock something loose in the mirrored tunnel. At this point it's best if the mirrors don't hang at odd angles inside the tunnels, burning more holes in the walls of the mirrored tunnel or falling and crashing down on the mirror-lined tunnel floor, causing a cascading disaster. For this reason I expect one side of every roof mirror to hang by metal strings in case of a disaster, while the other side melts loose from the roof. In this scenario the mirror hangs by the strings facing a 90 degree angle to the oncoming near-parallel sunlight. Assuming that the heliostat system properly shuts down in the event of a malfunction, the malfunction can stay limited until a repair crew arrives.
We may need to install tracks in the tunnel's ceiling that can support a mirror maintenance car.
E20. Replaceable reflector modules,
I recommend snap-in reflector modules for easy installation. Set up the post foundations, bolt the heliostats into place, snap in the mirrored reflector modules and power the system up.
I assume that heliostat targets each have two photocell units with their own computer chips, short-range networking units and power-saving batteries. Heliostat network units need to automatically find their nearby targets. If either the poles or the targets weren't properly installed, the heliostats need to correct for these imperfections as best they can. When the sun is shining the heliostats can rotate around in a search pattern to find their target photocells. The photocells will register 900% of normal sunlight whenever the sun is shining and the heliostat gets a focus on one of the photocells. Typically the photocells are located on two edges of a target. The system knows that when the concentrated sun is on a particular photocell, it's just barely missing the insect eye target on that edge. Using two photocells is useful because it's possible that one photocell unit will go on the blink.
Adding wires to a wireless network of heliostats and targets unnecessarily adds expense, so I recommend installing zero wires on the heliostat field. As I've noted before, the wireless radio frequency wavelengths of 10 or 20 years ago were pretty safe but modern wavelengths create an unnecessary health and legal risk to my way of thinking.
With photocells next to the solar target the heliostat's motors know when to turn the mirror. Also, in time the heliostat's software has learned with precision where to point its mirror at any time, tomorrow or next week.
The software needs to handle a number of contingencies. In the event of a malfunction or a human being or animal wandering in a restricted area, all heliostats need to point toward the sky, sending solar heat back into space. If the support post sinks a bit in the mud, the software needs to detect the problem. If one of the target's photocell units fail, the system needs to always recalibrate using the other photocell unit.
In the event of snow or freezing rain, the heliostats should protect their mirror surfaces from ice buildup. In the event of high wind gusts the heliostats should be edge-on to the wind direction.
E21. Microfocusing problems
At 42 degrees latitude the June 21 noontime sun arrives at an elevation of 70 degrees and sunset sun arrives at an elevation above the horizon of nearly 0 degrees. At noon the top of the heliostat is tipped backwards 35 degrees, moving the top edge of the heliostat backwards a meter from the target compared to sunset. The front edge is moved forwards a meter closer to the target. This means that at least some of the sunlight reflected at both extreme edges misses the target. Also, a slightly taller mirror is needed to reflect the same amount of sun at a more tipped angle relative to the target. With an insect eye target, some of the sunlight will go down the wrong insect eye, or some will miss the target entirely unless we have extra-wide eyes at the edges of the target. In any case we're going to send imperfect nearly parallel light down the light tubes. Given four targets for each heliostat arranged 60 degrees apart, we also have minor left-right focusing issues.
A heliostat stays with one of its four targets for about four hours. At the end of the four hour period the sun has moved 60 degrees and the heliostat has moved from pointing 15 degrees left of the target to 15 degrees right of the target. Then the heliostat quickly jumps 30 degrees to focus sunlight into the next target. In practice, one row of heliostats in a bright field would all take perhaps 15 seconds to jump from one target to the next, then the next row would turn, then a third row. We don't want double sun to go down any one mirrored tube at any time, and we don't want an intense dropoff in solar power with every heliostat changing at the same time.
E22. A self-adjusting heliostat tracking software algorithm
I'm a fan of self-installing solar tracking software. Given a small network of two photocells and controller chips on the edges of a solar target and a controller chip and two actuator motors on the heliostat, a 4x concentrating heliostat should, given full sun, run a two dimensional scanning pattern to find the photocells. When the 4x sunlight goes across a photocell it will signal a hit. The software will then define the two precise edges of the solar target using the two photocells.
Periodically as the sun moves across the sky, the heliostat will move its beam just a bit to see if it can just barely hit each of the photocells. At the end of the day the heliostat has recorded a fair idea of where to find the photocells at any particular minute of the day, with gaps in its knowledge where clouds have blocked out the sun. As the days go by the heliostat develops a pattern of precisely where to move the two actuators so as to land the sun's rays on the solar target. Quadratic extrapolation should calculate these minute by minute positions with accuracy even if the sky is mostly cloudy.
This extrapolation algorithm avoids an enormous amount of trigonometric calculations for locating the sun. The algorithm doesn't care about the latitude and longitude of the work site. The algorithm doesn't care if the heliostat has been set up on level ground or if the heliostat settles a bit crooked into the mud someday. The algorithm self-installs and it keeps working no matter what.
My goal would be to have photocell units with tiny batteries, with computer chips and with small wireless transceivers. The photocell units would attach to the edge of a window or other solar target without any wire connections. Heliostats would also optimally be self-contained units with zero connecting wires to worry about.
So-called 5G wireless technology uses wireless frequencies in the near-microwave range. What science knows about such frequencies says as clear as day that they may be hazardous to human health. 4G transmissions are better and 3G is better yet. Also, these wireless transmission technologies should be cheaper.
E23. Implications
Deliverable low-cost concentrated solar heat, combined with an ability to store great quantities of heat with low-cost thermal mass, changes most of the world's energy use. Most of the world's industrial processes involve heat. Heat boils water into steam. Low-cost solar heat can also be used directly in refrigeration processes.
We can think of brand new technology as high technology, as opposed to technology that poor villages can use, but high technology can get old pretty fast. Metal mirrors are a 2500 year old technology - the story of Archimedes destroying an enemy fleet with solar concentration is probably untrue.
Capturing this solar heat with mirrors means that the bare ground beneath the mirrors stays cool. In the end we're not adding to or taking away from the total solar heat already reaching the earth's surface. The sun shines down on the earth the same amount whether we have heliostats on the ground or not. Rather, we're relocating our incoming solar heat. At the far end of this road, heat concentration is liable to lead to warm air thermals transporting concentrated waste heat upward toward the stratosphere, and these thermal air currents make it easier for the planet's atmosphere to shed that heat. In sum, concentrating solar heat doesn't contribute to global warming at all.
For further reading: Generalized article on concentrated-solar-power, without any invention specifics. https://drawdown.org/solutions/concentrated-solar-power