B. Home Heat Storage
B1. Do rock beds work?
In theory, rock beds store solar heat in active solar air systems. Heated air from a solar heat collector would be forced by a fan through the rock bed, warming the rocks. Most rock beds were built underneath houses so that stored heat would rise from the rock bed into the house all night.
A rock bed with many thousands of rocks has a huge aggregate surface area for air-to-rock heat transfers. We don't want rocks so large that heat doesn't get transferred, nor so small that all airflow through the rock bed is choked off. A bed of rocks that fit through a 2” inch screen, generally 1” to 2” rocks, also known as 1 1/2” rocks, is a good compromise. My West Greenwich greenhouse uses about 4 tons of 1 1/2” rocks
In practice, most early rock beds grew toxic mold. The rock bed would work perfectly for one winter. Then summer would come around. The homeowner would stop sending hot air to the rock bed below the house. As weeks passed, the temperature of the rocks would drift down closer to the 55 degree F. temperature of the soil. Meanwhile, the homeowner would sometimes put moisture into her house's air from showering and dishwashing, and outside air on a summer night can see dewpoints above 70 degrees. When moist, warm air meets cooled rocks, moisture condenses on the rocks. That's when mold grows on the rocks.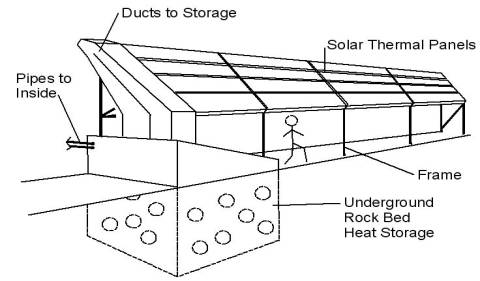
People can't live in enclosed air contaminated with toxic mold. I know of a family that inherited a gravel driveway because their next door neighbor threw out his moldy rocks from his rock bed.
Abandoned refrigerators can get moldy, but most working refrigerators in the 2020s seem to have solved their moisture and mildew problems. I believe that the issue of rock bed mold can be substantially solved by keeping the rocks constantly warm and/or constantly dehumidified, or by keeping the potentially moldy rock bed air 100% separated from indoor building air.
A rock bed will stay mold-free if the rock bed stays solar-heated for all 12 months of the year. So, I designed the Warwick rock bed to sit outdoors, two feet away from a building. In winter, a heat pump can draw on the rock bed's banked heat. In summer, the rock bed continues to collect solar heat from fan-blown hot air but the heat pump doesn't need to access that heat. At no time does moist air from the building get outside into the rock bed, and even if the rock bed were to grow mold, at no time could air from the rock bed get back into the building.
As with the West Greenwich greenhouse, a solar panel can power the fan whenever hot air needs to be blown through the rock bed.
I designed the rock bed so that the fan's air stream gets broken into extremely slow-moving air currents that travel through the rock bed. In this case the rock bed uses concrete blocks as air channels down into the rock bed and up from the rock bed. Air is blown through the system's solar heat collector, then down one set of concrete block air channels, through about 8 inches of rocks, up the next set of concrete block air channels and back to the fan in a closed loop.
3b2 show air in the rock bed
I designed and erected an aluminum and glass solar heat gathering system. The system sits two feet apart from the building. It has air gaps every 28 inches to spill wind overpressures. My sense is that hurricane-force wind gusts hitting the frame and the building's wall from the south will blow laterally up and over the roof in a way that imparts remarkably little uplift to the collectors. In any case, the frame supporting the collectors was designed by a reputable architectural firm and in the end I had to overbuild with heavier aluminum tubing than was necessary. And so, I suspect that a major hurricane would blow the building down long before it could blow my frame down.
B2. Frame safety
I wrapped rubber sheeting around certain diagonal frame beams because I didn't want anybody to bump their heads on those beams.
3b6 detail picture
As of 2022 the collector looks like it's in good shape. The roof over the rock bed seems to be holding up, with one potential roof leak. At one point I asked neighborhood kids not to sit on the rock bed roof.
3b7 me building the rock bed, picture, or did I put this elsewhere?
I made a fatal business error in building the prototype, but not an engineering error at all. I was building the system for a synagogue. It turned out that the synagogue had large staff expenses and so they were quietly burning through their last financial reserves. The synagogue suddenly went bankrupt before I could complete the system. Two years later the building was sold to a new religious group, and the new group had to go deeply into debt in order to buy the building. They would be happy to see their natural gas heating bill drop, but they couldn't contribute anything to the project.
Originally I had lined up an electrician who was willing to work pro bono on the project, but his life had changed two years later. One contractor wanted $8700 for electrical work alone because a heat pump is going to need a completely new electrical panel. I'd still like to prove the prototype but we personally never did have that kind of extra money.
Finally, I learned that most or all mini-split heat pumps aren't designed to push heat into a building if the outside temperature happens to be above 78 degrees F. Curiously, if the building is 100 degrees F. inside and the outside temperature is 100 degrees F., a heat pump will cool the building, but the process won't work unless the noisy end of a mini-split heat pump has been installed on the inside the building. So, I won't be able to add a commercial heat pump to the rock bed until I find out why standard heat pumps are purposely designed to fail at heating during hot weather conditions.
B3. Heating the West Greenwich Greenhouse
As has been previously discussed, the West Greenwich greenhouse stores heat in a rock bed underneath the floorboards and in the center of the greenhouse, in any spot that is a minimum of two feet away from the four walls. Whenever the sun shines a photovoltaic panel drives a fan, and the fan pushes the greenhouse's warm indoor air through the rock bed. We don't disconnect the PV panel from the fan in summer.
So far, heating results have been uniformly good. In spite of single pane windows and only R-10 insulation, the off-grid and zero fuel greenhouse stays roughly 25 degrees Fahrenheit above the average weekly outdoor temperature, which in Rhode Island has kept the greenhouse inherently frost-free all winter for four winters and able to grow warmer-weather crops in the shoulder months. The actual prototype agrees with my mathematical simulations that most of the solar heat doesn't escape through the ground when it’s placed into the center of the greenhouse, just above the topsoil beneath the greenhouse.
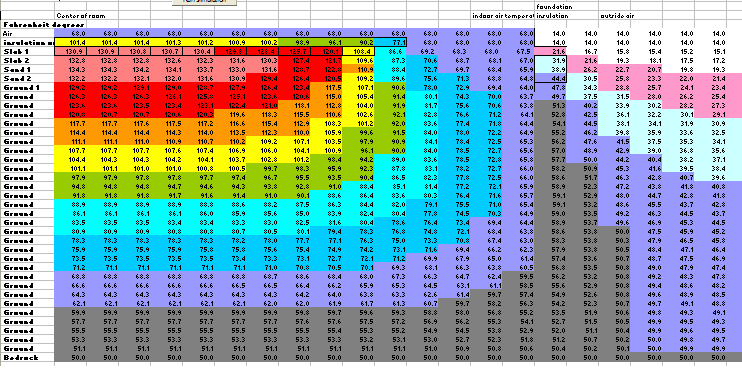
B4. Storing heat within the basement floors of houses
Most New England houses have concrete basements. I've also seen single-floor double-wide houses and big box stores that sit upon concrete slabs. In both cases the center part of the concrete slab, at least four feet away from each outer wall and the farther the better, should store multi-day heat fairly well.
The spreadsheet above depicts the flow of heat through the ground from a concrete floor to the outside in winter. Temperatures are in degrees fahrenheit. Solar heat is periodically added to the center of the basement floor, underneath an insulated mat. The part of the concrete floor nearest the wall doesn't have any heat input and doesn't have an insulated mat. I put insulation into the ground at the wall to a depth of 4 feet in this simulation.
Architects already understand that basement floors don’t lose that much heat straight down. If foundation insulation is used it typically insulates the outside edges of the basement floor or the basement walls near ground level. Heat might travel straight down a few feet into the ground below the slab, but once that ground gets warm the heat tends not to go anywhere else. Given a dry sand-like subsoil beneath the floor, heat stores well in the subsoil and leaks away quite slowly. A foot of dry coarse sand has an insulating R-value of about 7.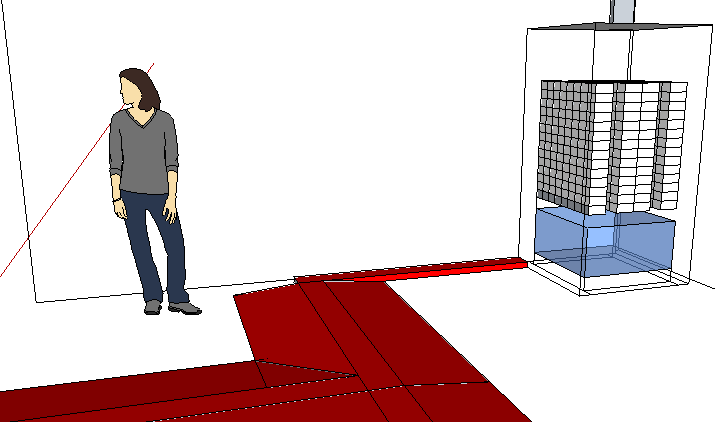
Fluid-based heat collection systems that can operate slightly above the boiling temperature of water are well-understood. To heat a basement floor I'd run a loop of flexible heat transfer pipes from a rooftop collector to the basement slab. I'd then want to cover the center of the basement floor with a strong, insulating two-inch thick mat that can contain one or more loops of flexible heat transfer fluid pipes. A thin metal mesh on the bottom of the insulating mat would optimize heat transfer rates into the concrete floor. The
mat needs to be able to withstand household foot traffic and wheelchair wheels.
Residential concrete has an R-value of .07 per inch of concrete. This R-value is helpful in calculating how quickly heat can be forced into the concrete from above, given a day or an hour of full sun. A quick spreadsheet analysis shows that the average basement floor can absorb sufficient heat from a good four hour or eight hour period of sun and heat.
In practice some heat will radiate laterally outward from the center of the concrete floor. The insulated mat should taper off near its edges to prevent tripping hazards and also to radiate a controlled temperature of heat back into the basement. No exposed portion of concrete floor should be able to burn exposed skin that might be touching the bare floor. The floor will tend to leak heat into the basement's air, leaking just a bit more heat during a day when heat is going into the slab. In the event of a winter electrical blackout the slab should keep heating the house for hours or for days. One of the above images shows all of the modular pieces that go into a mat. The other image shows a part of a fully assembled mat and the connector running to the heat appliance.
Here's an air-based version of basement floor storage. Solar heated air comes down one air duct, gets dispersed against the concrete floor and finally goes back up the other air duct in a closed loop. 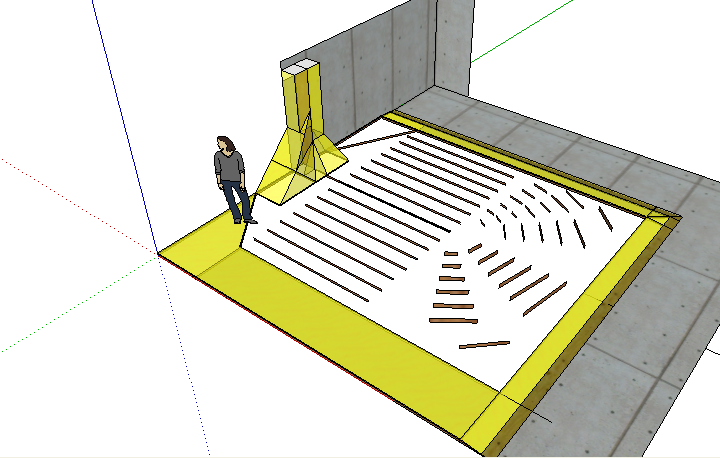
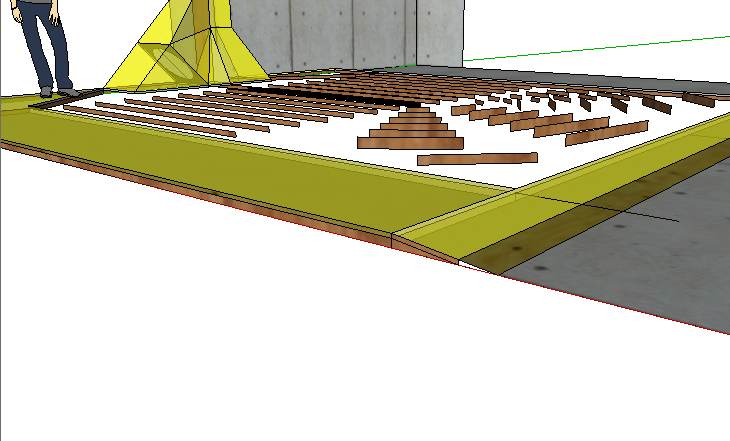
It remains to integrate this available thermal mass into an energy-efficient heating system with standard solar heat collection, with hot water storage and with a heat pump.
B5. A heat appliance as an alternative to solar hot water
Sand is a long-lived heat storage medium. Unlike water, sand doesn’t cause steam explosions, doesn’t crack pipes in freezing weather and doesn’t ruin any possessions when it uncontrollably leaks out of a pipe and fills the basement. Heat will easily conduct through air-sealed metal containers of sand laced with metal wires. To transport tons of sand into a heat storage appliance in a basement with low labor costs, a loop of sandblasting hoses can be snaked into a building and down staircases.
I first suggested using sand as a heat storage medium in the Helsinki Energy Challenge in the fall of 2020. Two Finnish researchers independently came up with a prototype that uses a silo full of sand to store heat in 2022.
draw the hose going down to the basement. I wish I could draw other humans too.
Current mini-split heat pump compressor units will stop heating if the outdoor temperature exceeds 75 to 78 degrees. We will need to re-engineer our heat pumps for our purposes.
Heat pumps have a second function: they cool a building in summer. It makes sense to use off-peak summer electricity and cooler morning temperatures to pre-cool either a basement floor or a coolness storage box.
We must not allow any cooling-related condensation because of toxic mold issues. Freezers once had frost buildup issues but modern freezers have no frost, so the problems of air moisture and condensation are obviously solvable. Multiple safeguards and regular inspections will be helpful. If we already require annual inspections of natural gas furnaces, why not require inspections of solar systems in order to prevent toxic mold growth?
Some basement floors occasionally flood. The mat needs to inhibit mold growth. A sump pump will help.
Some seasonal heat storage is possible given a drilled well and a low water table.
We need to integrate a centralized weather forecasting function and electrical grid supply/demand to optimize electrical grid summer and winter performance in rare weather contingencies. We hope to monetize electrical peak load electricity savings or “nega-watts.” We need to integrate consumer preferences into these heating plans.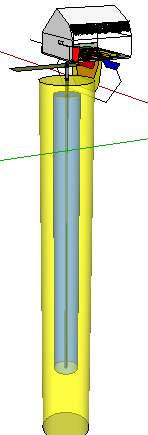
For further reading: Climate change: 'Sand battery' could solve green energy's big problem. https://www.bbc.com/news/science-environment-61996520
B6. Impact
Multiday basement heat and hot water storage should work for most of the Frost Belt, with perhaps a 90% reduction in lifetime heating fuel use for almost all existing houses and commercial buildings .
Based on my heat-storing greenhouse work I expect a high probability of success. Rooftop PV power will store solar heat. Heat storage should reduce the incidence of house freezing disasters.
A naturally warmer basement floor can add value to each building. It can reduce toxic mold and a musty smell in a basement. People may currently be urged to keep their home thermostats down to 68 degrees as a civic duty but young parents, arthritis sufferers and hot yoga lovers would all be happier if they had warmer floors.
Hyperinsulation is a competitor of sorts because every building has limited heating and cooling needs and insulation displaces a portion of those needs. Insulation has its limits, if only because people might leave a front door propped open to let stovetop smoke and toxic fumes out of a house in winter. At some tradeoff point, stored building heat will be more effective at reheating a building than hyperinsulating the building.
Air-based mini split heat pumps are energy-inefficient when the outside air gets below freezing. Geothermal systems based on drilling water wells are somewhat more energy efficient but installation is expensive. Drawing heat out of a warm basement floor will be optimally energy efficient.
The Drake Landing housing development in Alberta, Canada has been providing 97% of its own building heat through seasonal solar geothermal storage for a decade, and so seasonal hot water geothermal storage is our competitor for a certain size of district hot water heating. Repeating Drake Landing would cost an estimated $4 million up front for an optimal size of 200-300 homes. Our own proposed geothermal storage system, concept paper 2459-2288, is basement heat storage’s main competitor for supplying district heat.
Rock bed thermal storage will be a competitor when toxic mold buildup, dust and radon issues are solved. Our sand-based heat box is a working variant of rock bed storage.
B7. Storing coolness within a concrete floor
In the early 19th Century coolness was being seasonally stored, then shipped from New England to the tropics. Ice was cut from New England lakes in winter and stored all summer in well-insulated ice houses. The ice was loaded onto sailing ships, packed with sawdust for insulation and sold in the tropics for meat refrigeration and for cooling beverages.
Direct generation of coolness using solar heat is about 1/5 as energy efficient as generation of coolness with electricity. Often, combining a heat pump with coolness storage is best because the heat pump also heats in winter.
Then again, photovoltaic panels are often 1/5 as energy-efficient and possibly 1/10 as land-efficient as collecting direct solar heat. Furthermore solar heat can be seasonally stored and then used on demand, of course not in immediate proximity to a geothermal coldness storage site. So, the solar heat versus electricity argument isn't quite over, especially for the original generation of seasonally stored heat and coldness. District heating and district cooling will probably use water circulated through a seasonal geothermal storage area in order to heat or cool the water. Later on-demand transfer of the stored heat or coldness into hot water tanks, into commercial freezers or into HVAC systems is likely to require heat pumps.
home heat collection
B8. How not to solar-heat a house
I knew someone who installed a wall of single pane windows on the second floor of his house. That didn't work well at all. On a sunny winter afternoon his south-facing bedroom would get up to 90 degrees while the first floor of his house stayed pretty cold. Hot air rises, and even if the hot air is upstairs it won't travel downward by itself. Worse, by sunset his upstairs bedroom temperature dropped to freezing because single pane windows leak heat. Also, the upstairs bedroom's wooden walls didn't store much heat.
3a1 south side with old windows on second floor.
If nothing else my friend's solar experiment can serve the world as an early negative lesson in how to design solar heat.
- Use thermal mass to store solar heat, in order to displace fuel use after dark and on cloudy days. South-facing windows typically only displace 20% of a home's heating needs.
- Hot air rises. Thermal mass is heavy and so it typically doesn't belong upstairs. With solar air heat collection, use fans to move hot air downstairs to the thermal mass.
- Use insulation.
One more cautionary tale. We know of someone with a gravel driveway. His neighbor had an active solar air heat storage system with a rockbed that stored heat. In the first summer the neighbor turned off the solar heat capturing part of the system. The rockbed's internal temperature soon dropped to ground temperature. Moist air from the house eventually infiltrated down into the rockbed. The cool, enclosed rockbed grew damp. It grew toxic mold in that damp environment. That's when the neighbor threw his rockbed out. My friend inherited the rocks and that's how he paved his driveway.
3a2 moist air going down and toxic air going up
B9. Developing a specialized heat pump for this job
Your freezer is a heat pump. It's possible, if strange, to remove your freezer door, remove your house's front door, point the freezer out the front door, seal the door edges and turn the freezer on in midwinter. The freezer will extract heat from the zero degree midwinter air. The heat coils in back of the freezer will dump that tiny amount of heat into your house. If a neighborhood kid places a popsicle into your open freezer and then rings the doorbell, that's your problem.
3a5 A collage will do the job
An air-source heat pump extracts heat from the midwinter air and dumps it into your house. Heat pumps are especially energy-efficient if the outside air temperature is close to the indoor temperature. As the outside temperature drops, so does the system's energy-efficiency. If the midwinter air is below about 10 degrees to -15 degrees Fahrenheit, it makes more energy sense to run electricity through a heating coil to generate heat for the house.
A heat pump is reversible in summer. It can pump a house's indoor temperature down to 75 degrees and exhaust that heat outdoors.
Home heat pumps have often been engineered to handle certain specific temperatures. A hot water heat pump needs to get the hot water up to 120 degrees F or 49 degrees C. Most standard home heat pumps won't heat a building if the outside temperature is above 78 degrees F. This tidbit of information is important when using a solar-heated rock bed to heat a home.
Note: an electrical heating coil runs electricity through a coiled wire to create heat. That's not the same as a freezer coil which contains a certain fluid or gas.
B10. Heat transfer issues in an existing concrete floor
Heat flows slowly from hot spots to colder spots. If a radiator is full of 180 degree F. hot water and the room air is 66 degrees F., in time the radiator water will be down to 120 degrees and the room air will be up to 68 degrees. Given an enormous amount of time, the water and the air temperatures would become just about equal. However, in midwinter we can't wait an infinite amount of time or else the house will get seriously freezing. It's more practical to send the slightly hot radiator water back to the furnace or alternative heating system, re-boost its temperature to 180 degrees and cycle it back again for more heating.
3a3a and 3a3b, two thermometers, a radiator and air, before and after.
B11. With two or more cycles of heat transfer the maximum temperature drops each time
To deliver 120 degree F. solar hot water at the tap, the hot water tank may need to contain 140 degree hot water at the end of a sunny day and then the hot water tank will slowly cool off all night. To deliver 140 degree hot water at the tank, a 180 degree fluid (not water) may have to circulate through the tank's heat exchanger. To deliver 180 degree fluid, the fluid may need to be heated to 220 degrees in the solar heat collector on the roof.
Similar temperature loss provisions occur with solar hot air loops and heat storage.
3a4 draw two cycles
B12. Heat pumps plus solar storage
An air source heat pump always has free access to outdoor air.
3a6 I need a standard heat pump
A ground source heat pump takes heat out of the ground and pumps it into your house. If the ground underneath your house is, in Rhode Island, 55 degrees F., the heat pump doesn't expend that much electricity to move heat from 55 degree ground water to 68 degree indoor air. In summer, moving heat from 75 degree air to 55 degree ground water is a breeze. It's even possible on some days to directly circulate the 55 degree ground water through a 75 degree house's radiator pipes, cooling the pipes with the tiny amount of electricity needed to run the water pump.
3a7 illustration of a yard pipe
The trouble with a ground source heat pump is the extra cost of digging. One version of ground source piping digs up your entire yard to lay down a maze of pipes about 6 feet underground. Another version digs two water wells and ground water hopefully migrates from one well to the other, exchanging its heat or coldness with the ground.
3a8 Illustration of a two well system - get permissions?
Houses should combine solar heat storage and heat pumps. If a source of 100 degree F. heat has been stored on a winter day, a heat pump can quite efficiently heat the house on that winter's night.
B13. R-Values and BTUs
Long ago the English authorities established reasonable definitions of one foot and one pint, two commercially used measurements. By definition, one pint of water weighs exactly one pound at a certain standard temperature. In the 1700s Britain dominated world commerce. The U.S. continues to use the English system of measurement for the most part, and so I must describe BTUs and R-values for U.S. citizens who must use these units.
One British Thermal Unit (BTU) is defined as the amount of heat needed to warm one standard pint of water one degree Fahrenheit.
Given insulation with an R-value of R-1, one British Thermal Unit of heat will leak through one square foot of this insulation in one hour if there's a 1 degree Fahrenheit difference between inside and outside temperatures.
3a9 Draw one square foot of super-thin white insulation, a temperature of 68 degrees and 69 degrees, and an arrow going through the insulation.
For one square foot of R-10 insulation, 1/10 of a BTU will leak through each hour.
3a10 same picture with fat insulation.
If there's a 100 degree Fahrenheit difference on each side of one square foot of R-1 insulation, 100 BTUs will leak through each hour.
3a11 the 3a9 picture with 68 degrees and 168 degrees F.
Given a house with a certain R-value of insulation, heat loss per hour in BTUs can be calculated by finding the square footage of each of the house's walls and the roof, multiplying by the average Fahrenheit temperature difference between inside and outside and dividing by each wall's R-value.
3a12 R-10, 10' x 20' box, 20 degrees difference, show one wall 20 BTUs/hour and the roof is 40 BTUs /hr. Show measurements. Arrows show it going straight through. Dashed lines indicate sloped roof.
B14. Pass-through versus closed loop systems
Solar hot water is a pass-through system. Pressurized cold water comes from the city water pipes or from a well pump. The water gets heated, and then the water gets used once and directed down into the sewer system.
3a13 pass-through system. label everything
Solar heating of air is usually a closed loop system. Air is blown through a solar heat collector and then down to a rockbed which absorbs the heat, and then back up to the collector in an infinite loop.
3a14 label everything, closed loop system, show double duct
It's theoretically possible to take zero degree outside air, heat it in a solar collector, blow it down through a rockbed and then dump the still-warm air back outside into the cold, losing all that heat. That would be a seriously inefficient use of solar energy compared to building a closed loop. With the closed loop, 160 degree Fahrenheit air might be cooled to 100 degrees Fahrenheit by the rockbed, but then the solar collector would only have to reheat the air from 100 degrees up to 160 degrees, not from zero degrees to 160 degrees.
3a15 label it, single duct.
B15. Temperature limit testing
Every solar heat system will destabilize above a certain maximum rated temperature. It's important to know that all parts of the system won't overheat in all-day sunshine, even if an electrical system fails.
For my Warwick solar heat storage prototype, each solar hot air collector box was lined on three sides with R-6 insulation rated to 250 degrees Fahrenheit. The sun-facing side was a layer of aluminum painted with special solar heat absorbing black paint, covered one inch away with one layer of tempered window glass.
As a test I took a sample box unit, blocked it off on both ends to make it airtight and left it in full sun with a thermometer. It was 60 degrees F. outside that day with not a cloud in the sky. In 15 minutes the collector's internal temperature rose to 150 degrees F. and then the temperature stopped rising. At 150 degrees, new solar heat input was in equilibrium with total heat losses. I concluded that on a 100 degree F. day the internal temperature would never go past 190 degrees, so the insulation should never destabilize. Also, since the solar collector array would be installed at an angle that maximized heat in early March when the sun is about 40 degrees above the horizon at noon, the collector probably wouldn't get full sun on June 21 when the sun was 71 degrees above the horizon. Therefore the collector's inside temperature should never reach 250 degrees.
3a16 find that picture
It's possible to build a more heat-conserving solar collector, but then I'd still want to devise a method where, if the system's fan failed one day, my insulation wouldn't destabilize. Best guess, I'd have bi-metal openings in the heat collection units that would pop open with excessive heat buildup and would close after the excessive heat problem ended.
3a17a and b Bimetal open and closed
B16. Solar air heat collecting systems
The prime disadvantage of using air for transporting heat is the size of the air duct. A liter or a pint of warm air doesn't hold much heat. Retrofitting solar air ducts into an existing house often involves cutting holes in ceilings to install the air ducts, whereas flexible heat pump tubes can be snaked through small holes.
The advantages are many. Solar-heated air can be blown directly from a solar heat collector into a room. Unpressurized air costs nothing. No one ever has to check the air levels in the system. If warm air leaks out of an air duct it won't cause mold damage or wood rot to the house, nor will it poison anyone's pet, nor does anyone ever need to clean up a puddle of leaking air. Air can't freeze and burst pipes if the house ever freezes. Extremely hot air doesn't explode and air isn't flammable. Even a stray bullet from a drunken hunter isn't necessarily going to stop a hot air-based system from working. Dry air doesn't particularly react with most building materials including metals. Natural air contains no toxic fumes except in a few polluted cities.
A closed loop of hot air that has some air leakage is likely to evaporate any moisture that leaks into the system, and then the water vapor will leak outside the system. In this way a closed loop of hot air can inherently dehumidify the system.
B17. A Warwick, RI Experiment
3a18 the picture
Our Warwick, RI prototype was designed to control mold. We left a 24 inch gap between the rock bed and the building so that humid indoor air can never get into the rock bed.
Whenever the sun shines a photovoltaic panel turns a fan, which moves air in a closed loop from the rock bed up to the solar heat collection array an
d then back down to the rock bed. The hot air will typically evaporate any remaining moisture in the loop, and a bit of this hot air will leak out of the system, so that any liquid moisture that finds its way into the loop will soon be evaporated and will in time leave the system. In winter the dew point within the rock bed is likely to be around the freezing point even if the rocks are quite warm.
B18. The carport mount
Most solar heat collectors are mounted on the roof, within the roof or on the ground. This experimental collector was mounted on its own frame. We dubbed it the carport mount. Cars parked underneath would be a bit less likely to get hot in the summer sun.
3a19 focus on the illustration of a carport mount
In my citizen engineer's opinion, this particular carport mount design should withstand hurricane wind velocities beyond the velocity sufficient to knock down the building itself. When a southerly wind gust hits the wall of this building, wind overpressures build up on the building's outside south wall. Deflected air flows up and around the edge of the roof. The deflected airflow direction is more or less 45 degrees upward. This collector array is tipped 50 degrees upward, so the wind gust's airflow slides past the collector array on both sides of the collector array. 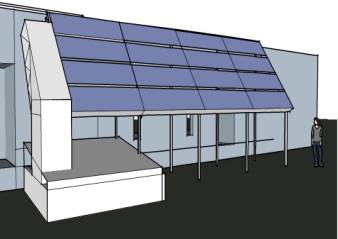
3a20 Draw the ideal airflow.
Westerly and easterly wind gusts will hit the array sideways. Northerly wind gusts coming off the roof above the top of the array will pretty much miss the array.
The array has air gaps built into it, so that any residual wind overpressures pushing the array up or down are somewhat evened out.
3a21 point out the three air gaps and arrows coming up out of it.
The array's frame was engineered to take 110 mph winds. On top of this we had to overbuild the frame because the mechanic needed to work with thicker metal posts.
We aim for easily replaceable and recyclable parts. We use safety glass. Always try to use heat-treated safety glass in any solar heat collection system because a combination of collected heat and a sudden downpour of cold hunderstorm rain can shatter normal glass.
In the end I'd say that the hot air collector looks nice but it was relatively expensive.
Alternative heat collectors
B19. An agricultural heat collecting system
Farmers are paying money for their propane heaters and for refills. Some farmers need heat to keep the animals' water tank from freezing. On one farm in Minnesota the chickens shivered so much in January that they had no energy left for laying eggs, so solar-heating a chicken coop can help with profits. Brood chicks need more heat than regular chickens. The farmer's own kids need warmth too. In this last case we would need to focus more on the potential for toxic mold issues.
Here is a chicken coop heater driven entirely by gravity. In fierce winter climates the chickens shiver so much that they don't lay eggs all winter. The sun's heat rises into the storage compartment and the storage compartment heats the chicken coop. It's simple to build.
A low-cost collector would be a layer of metal sheeting painted solar black, sitting on a hill slope. A layer of glass 1 inch above the sheeting would help, or even a racked array of inverted recycled clear glass jars, all the same size, might help with low temperature solar heat retention. If your system is too heat-conserving, a person or an animal might touch the system and get a burn,
On a slope, below the sheeting and above the bare ground hot air rises and cold air sinks. A thermal mass heat collection box at the top of the slope can take in and store the solar heat. Note that the ground below the solar collector gets relatively warm, and this warmth can rise into the heat collector after hours.
In the absence of a slope, a commercial attic gable vent fan with an attached photovoltaic panel will bring in the heat whenever the sun is shining, which is exactly when the heat will be available. Remember to remove the system's thermostat with a screwdriver. The fan is designed not to run when the air temperature is below 80 degrees.
Secure the sheeting to the ground with deep stakes.
3a26 show the stakes and sheeting
You'll need to leave perhaps a minimum of 3 inches between the ground and the metal sheets for air flow. The center parts of the metal sheeting might be propped up from below with extra glass jars.
Every summer, assuming that you don't need the heat, open a big air vent or two to let the system air out its heat. Covering the solar heat absorbing region with a tarp will also turn the system off, but the tarp might blow away someday.
B20. In-roof solar heat collection
Roofs are never designed to hold extra weight. Wood rot regularly reduces the amount of weight and uplift that an older roof can handle, until the roof is replaced or until it sometimes collapses. Many existing roofs can't support the additional weight of photovoltaic panels, solar hot water collectors or other fluid-based collectors. Also, certain partially shaded roofs aren't good for photovoltaic panels. A few tree branches in the way can seriously reduce total photovoltaic power, especially near sunrise and sunset.
For many houses I'd recommend removing shingles and roofing plywood from a steep south-sloping roof, adding greenhouse frames of tempered glass on top of the original roof rafters which have been painted white, and collecting heat in a deep box within the attic space.
The industry recommends using tempered glass, as opposed to ordinary window glass, on upward-facing solar heat collecting windows. Non-tempered glass has been known to shatter when the collector is still quite hot and when a sudden thunderstorm drops cold water or hail onto the upward-facing glass.
3a27 draw the roof, or find an already built (industry standard!!) house
Fluid-based solar heat collectors, where the fluid isn't water, are designed to capture solar heat at relatively high temperatures.
3a28 find an industry standard
I'll mention a competitor for 50 degree C water - digging a well two or six kilometers deep. There will be pumping costs. What if a pipe shatters 5 km down? What if there are lateral pressures on the hole? What if the output is limited after the immediate area around the well hass been cooled off to 30 degrees C?
B21. Canaries and air
A major issue with hyperinsulating all buildings is toxic building syndrome. As a rule, people buy brand new or refurbished houses and condos, and then specifically in the month of October lots of people move right back out again. That's because in October people start to shut their windows.
Exchanging air with the outside is one good way of removing some of the toxic chemicals such as toluene and formaldehyde that can build up in the indoor air of a well-sealed house. An air exchanger would also lower indoor airborne dust particles, humidity in the winter and the potential for airborne virus transmission.
A few people are violently allergic to peanuts. I remember a tragic newspaper story of a local college student who went to the wrong restaurant where the secret ingredient in her bowl of chili was peanut butter. That restaurant subsequently went out of business.
Other people are extremely sensitive to toluene gas fumes, to gasoline fumes, to pesticide fumes and hypothetically to nerve gas too. When inhaled, these fumes all go through the nose-brain barrier and cause trouble.
A natural pheromone smell from a possible lover can go right through anybody's nose-brain barrier in one inhalation and that causes them to unconsciously get all happy and interested. That seems to be the nose-brain barrier's primary Darwinian purpose. In any case, these fume chemicals first hit the brain's frontal lobe, where they bind to choinesterase within people's nerve synapses and basically shut the nerve synapeses down. Average airplane glue sniffers can feel drunk for 30 seconds on toluene fumes or they can sometimes pass out. No, this isn't good at all for the glue sniffer's brain cell development.
Hypersensitive people have dramatically less available cholinesterase, and so a dose of fumes that wouldn't bother most people will get them drunk and then sick for a few days. My wife is one of these people. She's a canary. If she keels over, the rest of you should get out of that room quickly (and somebody carry her unconsious body out with you as you leave please).
Yes, it's known in the canary community that methane gas heaters and stoves affect both asthmatic people and chemically sensitive people. It's probably medically correct to have nobody occupy a building or a room if the canary can't be there, and it's sociologically correct to avoid gratuitously excluding a person with a disability from your in-group. I can think of multiple instances where Friends moved a workshop because the space had fumes that most people's noses didn't detect.
In any case, I recommend combining stored solar heat with a heat pump to displace 100% of natural gas heat. Hyperinsulating a building sounds nice but it can lead to toxic house syndrome if you're not careful.
B22. Mold
25 years ago I defrosted a freezer every four months. Two years ago we had a malfunctioning freezer that needed regular defrosting. Today our new freezer doesn't need any defrosting at all. I say this because the biggest engineering obstacle for solar heat storage and for solar-based coolness storage is moisture buildup. Take heart, because the refrigeration industry once faced this same moisture buildup problem and they effectively fixed it.
I combine a mini split heat pump with a rockbed to deliver both heat and hot water on demand. The goal is inexpensive 90% solar, 10% renewable electricity to run the heat pumps and 0% fossil fuel or biofuel, with a heat setback at night for smarter energy performance and then a reliable 68 degrees by 6:00 a.m.
I expect that the new renewable standard will be 90% solar. If you plan on purchasing or building an edifice that achieves far worse than this energy standard, then you will have stuck yourself with a long-term unprofitable building and you'll have to pay the stiff carbon tax. Renters want that climate change cachet, which happens to be good for own their bottom line, and that's going to permanently affect your rental prices and the building's eventual resale value.
B23. Photovoltaic power and dirty electricity
an issue: smart meters give off near-microwave electromagnetic radiation - people get sick.
solution: fewer bursts, bursts only when asked, move back toward 3G and 4G wavelengths, don't send as much information, get wired, use the electric wires!
HVDC lines vs. killer-watt lines.
Sidebar: making smart meters far safer.
B24. Canaries and wifi
Wifi meters should pulse only when told to pulse. once a day vs. once every three seconds.
Our society mustn't use near-microwaves except perhaps to cook food. Radio waves work.
We can design individual safety clothing, similar to Faraday bags
Why would you want to be tracked by your smart phone anyway?
Cables are more dependable.
B25. HVDC lines vs. 220 Kv HVAC lines
If you hold a 4 foot long fluorescent light bulb underneath an average 220 kilovolt High Voltage Alternating Current electric line at night, the bare bulb will glow all by itself. People have posted videos of this effect on youtube.
This means that if you're standing underneath a 220 kilovolt HVAC line, you're probably glowing a bit too. Farmers complain that the lines are giving leukemia to their cattle. Birds are attracted to high voltage lines in the winter because of all the waste heat that these lines give off. High Voltage AC lines waste power.
The newer High Voltage Direct Current lines are notably safer for people and animals, and they also can carry electricity long distances without power losses. It's now possible to ship electricity hundreds of miles from a power source to an energy storage site and on to the consumers. Ask your local utility which lines they are proposing. If they don't give you a straight answer it's probably because you won't like the answer one bit.
Solar farms and rooftop solar installations often use smart meters. Smart meters and 5G Ultra broadcasting stations can emit near-microwave radio waves that are medically hazardous for the canaries living among us. A high-quality developer will take steps to reduce this pollution. I've seen one report that about 25% of the population suffers minor medical issues such as sleeplessness and light headaches, while a very few people get seriously ill or die. This compares somewhat to gratuitously killing all people with violent peanut allergies in the name of somebody's profits.